5G radiation
In addition to the promise of faster data transfer rates and lower latencies, 5G is often talked about because of another aspect: The concern about health disadvantages from 5G radiation. These concerns range from the obviously crazy conspiracy theories of 5G as the cause of Covid-19 to the serious reissue of concerns about cell phone radiation, which had been on the minds of many since the 1990s.
In addition to the promise of faster data transfer rates and lower latencies, 5G is often a hot topic because of another aspect: The concern about health disadvantages from 5G radiation. These concerns range from the obviously crazy conspiracy theories of 5G as the cause of Covid-19 to the serious reissue of concerns about cell phone radiation, which had been on the minds of many since the 1990s.
What's the concern about 5G radiation? Is it dangerous? How is 5G radiation to be assessed in comparison to the older mobile radio generations - and how in comparison e.g. to radio stations and similar radiation sources?
To be able to judge this, we have to dig a little deeper into the topic.
5G - what is this anyway?
The abbreviation 5G denotes the so-called 5th generation of mobile radio standards. These standards essentially specify the wavelengths and transmission strength with which the cell phones and base stations are allowed to transmit by international bodies. The second important requirement is transfer protocols, i.e. how sender and receiver communicate with each other.
Once upon a time there was an A network
The first mobile radio generation, in Western Germany that was the A, B and C networks, still worked purely analogously. It was introduced in the 1980s. The wavelengths used for the A and B networks were in the 2m range. This is roughly the range in which FM radio stations are also broadcast and received. The C network was assigned a wavelength of 70cm.
These networks were still very cumbersome (e.g. in the A network, a switching center still had to set up the connection by hand) and were already at their capacity limit with a few 10,000 participants in Western Germany. Because there were only a few receiving stations, the handsets also had sporty transmission powers of up to 30 watts.
The most modern German network of the first generation, the C network, had the major disadvantage that the standard used, C-450, was not compatible with the standards of the neighboring countries. Roaming, i.e. using the mobile device in another country, was therefore not possible.
2G, 3G, 4G, 5G
That was one of the points that should be improved with GSM, the new standard for the next generation of mobile communications. In Germany, GSM was the basis for the D and E networks that were introduced in the early 1990s. The GSM standard enables different frequency ranges. A frequency range of approximately 900 MHz is used in Europe, Africa, the Middle East and parts of Asia. GSM is retrospectively regarded as the second generation of mobile communications and is therefore now abbreviated as 2G.
A trend can now be recognized: the newer mobile radio technologies are using ever higher frequencies. Higher frequencies make it possible to transmit more data in the same time or to be able to operate more mobile devices from a single base station. This enabled better sound quality at first and later also data transmission. But you also pay a price: the range is reduced. You may still be familiar with this from analog radio: An FM transmitter in the car radio initially sounds very good, but catches e.g. after just a few kilometers you start to rustle. A long-wave transmitter, on the other hand, does not sound stunning from the start, but can still be heard even after hundreds of kilometers.
Likewise, the first cell phones only required a comparatively few receiving stations distributed throughout the country. In view of the few users, only this made the undertaking affordable. Later there were far more interested parties, so it was worth installing the appropriate infrastructure of thousands of transmission masts. This in turn enabled the mobile devices to function with less transmission power - with 2G it was only 2 watts instead of 30 in the A network. This requires less battery capacity and brought the devices from the trunk into your pocket.
This trend continued similarly: For the third generation, the standard is called UMTS, the frequency bands were auctioned to 8 companies in Germany in 2000. These 3G frequency bands are all around 2100 MHz (but the range of possible frequency bands start at 700 MHz). At the same time, the maximum permissible transmission power of the mobile devices decreased again: With 3G, it is only 0.25 watts.
This was followed by an interim solution called LTE (Long Term Evolution), which, although it was often marketed differently, still belonged to the third generation of mobile communications. Only with the further development of LTE Advanced is 4G also spoken in technical circles. With the new standards, the upper limit of the possible frequency bands has shifted again: for 4G it is around 2600 MHz. The permissible transmission power is 0.2 watts.
Which brings us the the present, where the fifth generation has arrived: 5G. With 5G, the potentially usable frequency bands have been significantly shifted upwards: They can be up to 40GHz, and extensions up to 80 GHz are already planned.
Wavelength vs. Frequency of electromagnetic waves
Electromagnetic waves can be described in two ways: The wavelength describes, as the name suggests, the length of a complete wave, i.e. an oscillation that starts at zero, deflects upwards, deflects downwards and ends up at 0 again:
The frequency tells you how often this vibration happens in a second. Electromagnetic waves propagate at about the speed of light (it depends a bit on the material), i.e. 300,000 km / second. A wavelength of 2m, as used in the A and B networks of the first generation of mobile phones, fits 150 million times in the distance that the light travels in one second. The frequency unit is Hertz (abbreviated Hz) - the wavelength of 2m corresponds to a frequency of 150MHz.
Radiation is not the same as Radiation
Particle radiation
So far, so good - but what kind of radiation is it anyway? Without going into too much detail here, a rough distinction can be made between particle radiation and electromagnetic radiation.
Particle radiation arises from the radioactive decay of atoms. Either parts of an atomic nucleus (alpha radiation or neutron radiation) or the electrons from the shell (beta radiation) are emitted. In weak form, we are exposed to alpha and beta radiation through natural decay processes in the environment, but this is not a health problem in most parts of the world. Most of the neutron radiation reaches Earth through cosmic radiation - but we are protected from it by the Earth's magnetic field. Apart from the targeted medical use, particle radiation in higher doses is, in practice, something that is definitely not health-promoting.
Electromagnetic radiation
Electromagnetic radiation does not send out particles. Every day we are surrounded by electromagnetic radiation in very different wavelengths - from waves with a length of several meters to extremely high-frequency radiation with wavelengths of a few nanometers.
Electromagnetic radiation is important for life on this planet - so important that we humans have developed two sensory organs to be able to perceive it: an organ for wavelengths of approx. 400 to 780 nanometers (nm), and an organ for wavelengths of approx. 1-10 micrometers (µm), i.e. 1000-10000nm.
Of course we mean our eyes and our skin. The largest source of natural electromagnetic radiation is our sun. And that in turn radiates the most in the range of approx. 300-1000nm. That is why our most important sensory organ is designed to be able to perceive precisely this frequency range in detail. The area of electromagnetic radiation that we can see we are calling light. Radiation with waves longer than light we cannot see with our eyes, but with our skin: it is infrared or heat radiation.
Longer wavelengths than the visible light
Light and infrared radiation are identical except for the different wavelengths - they are simply electromagnetic radiation.
Infrared radiation is the area of electromagnetic radiation that borders the light at the long-wave end. Some animals, such as some snakes, can still "see" parts of this spectrum and use this ability for orientation. Terahertz radiation, which has wavelengths of up to 1 mm, is even longer-wave than infrared. While we have been producing light and infrared radiation artificially for thousands of years, first with campfires, now with LEDs and heat lamps, the terahertz radiation has so far been neglected by our species. The situation is different with the adjacent, longer-wave range: microwave radiation. With wavelengths in the centimeters and frequencies in the gigahertz range, it plays an important role in the modern world. In addition to the microwave in the kitchen, forms of medical therapy, radar systems in aviation, shipping and, more recently, autonomously driving cars, this is also the area in which all cell phone frequencies from the first generation are located, from the C network in the 1980s - up to and including 5G.
Shorter wavelengths than the visible light
On the short-wave side of light are all the areas of EM radiation that are known for their health risks: Next to the deep blue we humans can still see, the ultraviolet radiation or UV light begins. UV radiation has wavelengths from approx. 10nm to approx. 400nm. The strongest natural source of UV radiation is our sun. They can no longer see human eyes (by the way: this is also the reason why sunscreen looks transparent to us!), But e.g. Bees' eyes have developed in such a way that they can still perceive parts of this spectrum.
UV radiation is used for disinfecting materials. It also plays a very important role in spectroscopy: With the help of spectrometers, substances can be distinguished very precisely based on their reaction to UV light. This is used to e.g. check the bacterial contamination of food samples or to decode the composition of an unknown material.
The shorter the wavelengths get, the more energetic they are, i.e. X-rays have higher energy density than UV light. In addition to being used as a medical imaging technique, they are often an undesirable by-product of other technologies, such as military radar equipment or early generation CRT televisions. Gamma radiation is even more short-wave and therefore more energetic. It occurs in radioactive decay processes. Gamma radiation is extremely short-wave, its wavelength is given in picometers (pm), i.e. thousandths of a nanometer. Gamma radiation is used medically: in radiation therapy, for example, it is used to kill tumor cells. There are also spectroscopic methods that work with the spectrum of gamma radiation.
Harmful effects of electromagnetic radiation
Why is it now dangerous to the "left" of visible light, but largely harmless to the "right"?
The reason is the photons - the elementary particles emitted by electromagnetic radiation. Photons are also called light quanta or light particles. Photons do not weigh anything, i.e. they have no mass. But they do have energy, and this depends on the frequency with which the photon vibrates: the faster the vibration, the more energy the photon contains.
The faster a photon vibrates, the less distance a beam can travel before the vibration has returned to the starting point - a faster vibrating photon therefore has a shorter wavelength. Many complete vibrations then fit into the distance that the beam travels in one second - a faster vibrating photon therefore has a higher frequency.
Ionizing radiation
Above a certain amount of energy (in electron volts, eV), i.e. at very small wavelengths, the moment is reached when the photon carries such an amount of energy that it can release an electron from its path when it hits an atom. This turns the previously neutral atom into a positively charged ion, which can now interact with its surroundings - a free radical has developed.
Radiation which, because of its small wavelengths, contains enough energy to accomplish this removal of electrons is called ionizing radiation. Ionizing radiation starts at wavelengths of approx. 250nm - i.e. with UV light.
This is also the way that UV light can cause skin cancer: individual atoms are ionized, and this leads to interactions with other atoms and molecules within a cell. If the damage is large enough, the result is a mutation in the genome or even cell death.
Even shorter-wave radiation is even more energetic and can therefore lead to cell damage more quickly. Therefore e.g. X-rays are used so sparingly in medicine.
Non-ionizing radiation
Longer-wave radiation is no longer energy intensive enough to do this. To be more precise: With long-wave radiation, the atoms would have to have such weak bonds that they would disintegrate all by themselves in our normal environmental conditions.
Longer-wave radiation still has effects: depending on the wavelength, its energy is absorbed by different atoms or molecules, and this is equivalent to heating. The microwave oven makes use of this effect.
5G radiation: not ionizing, but potentially heating
The frequencies with which 5G radio works are therefore still very far (about 10000x) below the threshold of ionizing radiation, even in the planned expansion stages of 80GHz.
Radiation from 5G cell phones
Undesired effects can really only arise due to warming of tissue. This is the effect that microwave ovens or laser cutters make use of. Although the maximum permissible transmission power for 5G mobile devices is specified at 0.2 watts, this radiation does not affect the whole body evenly, but only areas very close to the cell phone, and different devices differ in their technical details, which makes them difficult to compare. For this reason, the SAR value was introduced as a standardized comparison option. This value, which is defined in the European standard EN 62209-1, describes upper radiation limits when operated at the ear, and also the exact way in which the radiation power is measured. The permissible upper limit is 2W / kg body weight, and practically all devices on the market fall well below this value.
Radiation from 5G base stations
Let us consider the other side of radio communication: the base stations. The base stations usually work with 50W input power, but mostly do not emit perfectly shperical, but in a directed way. The effective radiative power (ERP) can be up to one kilowatt. For comparison: radio long-wave transmitters or television towers work with input powers of up to 500 kW. Since electromagnetic radiation basically spreads in all directions and the 5G frequencies in particular are strongly absorbed by many particles in the environment (moisture in the air, trees etc.), the radiation power actually arriving depends very much on the distance to the base station.
The Research Foundation for Electricity and Mobile Communication (FSM) at ETH Zurich has repeatedly measured the strengths of various sources in an urban environment. The result: approx. 1/3 of the radiation arriving at a person comes from cell phone base stations and other people's cell phones, a third are radio and television transmitters, and the last third are made up of cordless phones and wifi access points (source).
Other possible effects of cell phone radiation
Potential effects beyond the heating of tissue have been researched using various approaches for several decades. Similar to the influence of blue light on sleep, where high doses before bedtime have an influence on the serotonin levels, but sleep behaviour itself was practically identical, there are EEG-detectable influences on the non-REM sleep patterns - but sleep as such was again indistinguishable from sleep without exposure to a strong EM field (source).
Cell phone radiation and cancer?
Even if the formation of free radicals by ionizing radiation as a cause of cancer is excluded, because the mobile radio frequencies are very far away from the frequencies of ionizing radiation, this is not proof of the non-existence of an influence - because such evidence is empirically not possible in any research field in the world. That is why there were a number of larger surveys that examined the connection between cell phone radiation and cancer.
Of particular importance here is a Danish cohort study that has accompanied the majority of all cell phone users for several decades. It is particularly valuable because it a) comprises a substantial part of the cell phone users, i.e. it wasn't the case that at-risk parts of the population were somehow systematically excluded from the study b) it was a proper field study, not only under measuring under unrealistic laboratory conditions, and c) the observations took place over an extremely long period of time - they started in 1982 and lasted until 2007.
The result: No increased cancer diseases could be detected (source).
Conclusion: Does 5G radiation make you sick?
Most likely not. There will certainly not be any strong effects: the frequencies used have been in use for decades, sometimes with thousands of times the radiation power (e.g. from analogue radio and television towers) - without any demonstrable effects.
In addition, the range of 5G base stations and mobile devices is very short because the radiation is strongly absorbed by the environment. The 5G network will therefore consist of many weak stations instead of fewer strong ones. This will further reduce the individual maximum exposure.
Nevertheless, as with all technologies, the precautionary principle should be applied here: putting the cell phone on your bedside table into flight mode only takes a second and has no further disadvantages - so you shouldn't get out of that habit even with the 5th generation.
You might also like
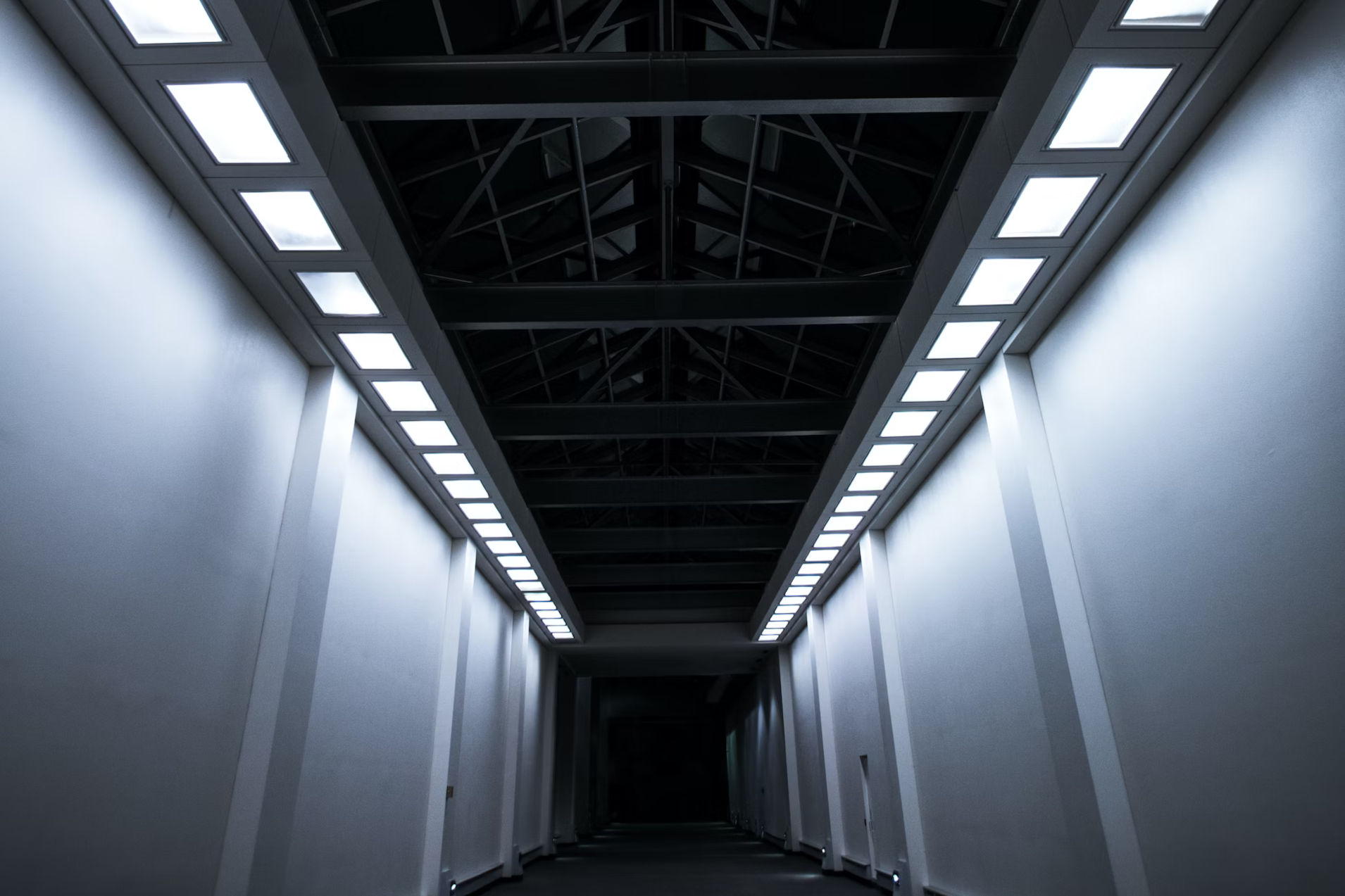
Nits, Lux, Lumen, Candela - calculating with light and lighting
Luminous flux, luminous intensity, luminance and co. - when is which quantity used and how can they be compared?